Tissue engineering strategies for breast reconstruction: a literature review of current advances and future directions
Introduction
Background
Breast cancer is a significant health concern among women in the US, with over 280,000 new diagnoses in 2022 (1). Surgery is the most common treatment approach (2). Mastectomy, which involves removing the entire breast tissue, is a standard component of treatment for many patients with breast cancer. In contrast, nipple-sparing mastectomy (NSM) is a surgical technique that involves removing the breast tissue while sparing the nipple and areola complex (3). NSM is not considered a safe option for all breast cancer subtypes and cases, particularly those with multifocal tumors or hormone receptor-negative/ERBB2-positive subtypes (4,5). In such cases, total mastectomy remains the gold standard therapy. For both these approaches, reconstruction is an option proposed by the plastic surgeon and is chosen by up to 40% of the patients (2). Adjuvant radiotherapy and chemotherapy can obstruct reconstructive surgery by damaging the remaining soft tissues. Different techniques are clinically used to achieve total breast reconstruction while facing its challenges.
Implant-based techniques consist in reproducing the breast volume by using a silicon implant. In contrast, autologous techniques recreate the breast shape using various flaps (free or pedicled, fascio-cutaneous or musculocutaneous flaps) or exclusive fat grafting. Implant-based and autologous techniques can also be combined. Each of these techniques has advantages and downsides: Implant-based reconstruction following mastectomy is easy to perform, with moderate technicity (no fine dissection or microsurgery) and short operative time but is limited by the poor skin laxity and the long-term cosmetic outcomes due to capsule contracture (6). Moreover, multiple surgeries are needed due to the limited lifetime of current silicon implants (7). In contrast, autologous flaps provide a natural result that can be stable in time and avoid any foreign materials with all linked disadvantages (8). However, it requires a high degree of technical skills for the flap and pedicle dissection, longer surgeries, and may still need improvement despite recent microsurgery advances. Nipple-areolar complex (NAC) reconstruction has improved with the advent of 3D tattooing, but local flap techniques still need to provide a long-term projection of the nipple (9,10). Overall, all of these techniques still fail in recreating an identical breast and fully restoring the femininity of affected patients.
Rationale and knowledge gap
The importance of this article for the readership lies in its role in highlighting the evolution and potential of tissue engineering in the field of breast reconstruction. Tissue engineering, a concept introduced by Joseph Vacanti and Robert Langer in the late 1980s (11), offers a multidisciplinary approach that combines engineering, biology, and medicine to develop biological substitutes for damaged tissues and organs. While the initial applications of tissue engineering focused on cartilage regeneration (12,13), the rapid interest of breast surgeons in the unique properties of acellular dermal matrices (ADMs) and fat tissue processing opened up new avenues in breast reconstruction. Over the past two decades, numerous innovative concepts and applications of tissue and bioengineering have emerged in this field. By exploring these advancements, this article aims to provide valuable insights to readers, including researchers, clinicians, and medical professionals involved in breast reconstruction. It serves as a knowledge resource, showcasing the progress made in tissue engineering techniques and their potential impact on improving outcomes in breast reconstruction procedures.
Objective
This work aims to review all the different applications of tissue and bioengineering in breast reconstruction and describe how surgeons can use it to improve the outcomes of these complex surgeries. We will explain the various techniques for each purpose: improving the skin envelope, increasing fat grafting trophicity, enhancing the breast shape and volume using 3D printing, and upgrading refinements through NAC reconstruction. We present this article in accordance with the Narrative Review reporting checklist (available at https://atm.amegroups.com/article/view/10.21037/atm-23-1724/rc).
Methods
We performed a literature search on PubMed and Google Scholar Databases between April and June 2023. The search strategy (Table 1) excluded no species to include future directions. Articles published before 2000 and articles in languages other than English and French were excluded. To allow for a better focus on each sub-objective, we performed different searches for each purpose, using the following combination of keywords and Boolean operators:
- Breast reconstruction AND Skin AND (Tissue Engineering OR Dermal Matri*);
- Engineering AND (Fat graft* OR Lipo*) AND Breast reconstruction;
- Engineering AND Breast reconstruction AND (Printing OR Scaffold);
- Engineering AND Nipple reconstruction.
Table 1
Items | Specification |
---|---|
Date of last search | June 6th, 2023 |
Databases and other sources searched | PubMed, Google Scholar |
Search terms used | (I) Breast reconstruction AND Skin AND (Tissue Engineering OR Dermal Matri*) |
(II) Engineering AND (Fat graft* OR Lipo*) AND Breast reconstruction | |
(III) Engineering AND Breast reconstruction AND (Printing OR Scaffold) | |
(IV) Engineering AND Nipple reconstruction | |
Timeframe | Published after 2000 |
Inclusion and exclusion criteria | Inclusion criteria: articles reporting on tissue engineering or bioengineering-based techniques for breast reconstruction |
Exclusion criteria: language other than English or French | |
Selection process | Selection was independently conducted by the two first authors (Berkane Y and Oubari H), and consensus was obtained by the senior author (Lellouch AG) if needed |
This article used the Evidence-Based Veterinary Medicine Association (EBVM) Toolkit 14 checklist for narrative reviews as a quality assurance measure. Additionally, we used the Scale for the Assessment of Narrative Review Articles (SANRA) tool [2019] to self-assess our methodology (14). It is noted that these guidelines were not used to assess the quality of the included articles but rather to ensure the robustness and credibility of our research process.
Literature review of tissue- and bio-engineering approaches in breast reconstruction
Breast reconstruction aims to restore volume, texture, shape, and symmetry. Plastic surgeons use various techniques to achieve this, and engineering-based approaches have allowed significant advances (Figure 1).
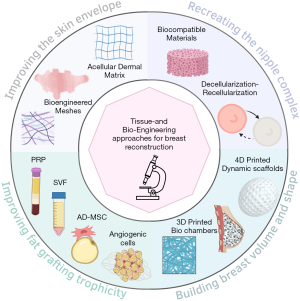
Improving the quality (thickness and elasticity) of the skin envelope
ADMs have been extensively used in breast reconstruction for several decades. ADMs are skin matrices processed to remove all cells and antigenic elements, with a threshold of less than 50 ng/mg of tissue of double-stranded DNA (15). These matrices can be sourced from humans or animals (16-18) (Figure 2). They consist of collagen, elastic fibers, and other components of the extracellular matrix, such as fibronectin, laminin, and hyaluronic acid, which provide support for the recipient patient’s cells. It has been demonstrated that acellular matrices undergo neo-vascularization and recellularization by host cells (19-21). In breast reconstruction, ADMs are used to enhance skin coverage, particularly after the placement of a silicone implant or tissue expander (4,22). Another goal is to reduce the risk of capsule contracture, a significant complication of implant-based surgeries, leading to a substantial decrease in the long-term cosmetic result (23). Some authors have described the risks associated with using ADMs, including infection rates, skin blisters, and overall complications (22,24). Some concerns were initially raised related to red breast syndromes found to be linked to ADMs by reconstructive surgeons (25,26). However, the probable bacterial origin of this syndrome has led to solutions improving the outcomes (27-29). As an interesting alternative, Gentile et al. described the use of titanium-coated polypropylene mesh in conservative mastectomies and pre-pectoral breast reconstruction (29). Their results showed better cosmetic outcomes when compared with tissue-expander-based techniques using the Breast-Q form.
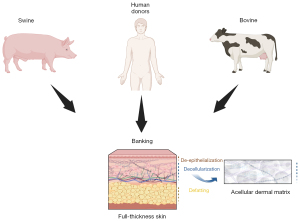
A retrospective review conducted over ten years at the Massachusetts General Hospital included more than 3,000 patients undergoing breast reconstruction (4). One aspect of their analysis compared the outcomes of pre-pectoral implant reconstruction with ADM-enhanced coverage versus subpectoral techniques, where the implant is placed partially under the muscle. The study found a lower rate of nipple necrosis in the ADM group, with similar rates of infection, explantation, seroma, and overall reconstruction failure compared to the subpectoral group. Additionally, when comparing ADMs with synthetic mesh products, the study found higher complication rates with synthetic mesh. This highlights the advantages of using tissue-engineered biocompatible solutions instead of synthetic products. A study by Graziano et al. (30), based on the American Cancer Society database, focused on national outcomes of prepectoral breast reconstruction. They found that most (55%) of direct-to-implant reconstructions utilized ADM. Despite a slightly higher reoperation rate, the study reported a lower infection rate in the ADM cohort. However, regression analysis showed that caution should be exercised when using these scaffolds in patients with insulin diabetes, obesity, or active smokers. The significant limitations of this study were its retrospective design and short follow-up period of 30 days. The CARE trial (31), supported by Allergan (AbbVie, Chicago, IL, USA), is one of the first prospective studies with long-term follow-up. It revealed a twofold decrease in capsular contracture rates with ADM at the five-year mark and lower rates of implant malposition. These results were consistent in revision surgeries, highlighting the ability of ADMs to improve tissue health and provide stable, long-term outcomes. Forsberg et al. (32), followed by Ibrahim et al. (33) performed two interesting studies assessing the aesthetic outcome following tissue expander/implant-based breast reconstruction with and without ADMs. They found improvement in the overall aesthetic result, as well as in breast contour, implant placement, lower pole projection, and inframammary fold definition, as evaluated by five blinded plastic surgeons. The recent BROWSE multicenter study (34) carried out over a decade brought more evidence supporting improved aesthetic outcomes and reduced capsular contracture with prepectoral implant with ADM reconstruction compared to submuscular. The meticulous methodology, using the strongly validated BREAST-Q questionnaire (35), provides solid evidence supporting ADMs. Other authors have also supported these findings, emphasizing the advantages of ADMs in terms of safety (31,36), objective aesthetic outcomes (33), prevention of capsule contracture (31,33), and postoperative pain reduction.
Furthermore, the development of ADMs has facilitated the rise of prepectoral reconstructions, reducing the morbidity associated with subpectoral implant surgeries while maintaining safety and potentially improving aesthetic outcomes (32). Finally, while ADMs do add certain costs, de Blacam et al. (37) showed that single-stage implant procedures with ADMs are less expensive than tissue-expander-based approaches. On the other hand, as demonstrated by Bank et al. (38) in 2013, the use of ADM reduces the number of visits required for reconstructions with high-volume implants. A cost-effectiveness analysis by Jensen et al. demonstrated a positive impact of its use in breast reconstruction, which was confirmed by Krishnan et al. (39) found an increase in quality-adjusted life years.
Overall, ADMs have shown solid evidence as an optimal support for implant-based reconstructions, with excellent safety.
Improving fat grafting trophicity
Autologous fat grafting has several beneficial characteristics, including lack of immunogenicity, safe and simple procedure with low complication rate and positive results, low cost, and easy usability (40-43). For this reason, fat grafting has become a standard of care in breast reconstruction for patients who do not wish to go through a complex surgery such as flap reconstruction or are reluctant to the idea of a foreign material such as a breast implant. Fat grafting has shown excellent natural cosmetic results when compared with implant-based breast lifting (41,42). If implant-based solutions provide immediate results with long-term volume preservation, some authors emphasized more natural aesthetic results with fat transfer (43). However, the volume loss after fat grafting is a significant issue, with a 30% to 40% loss of the initially grafted volume, according to the authors (44,45). Different solutions to improve fat survival have emerged thanks to recent bioengineering advances.
The first approach consists of platelet-rich plasma (PRP) enrichment. Some studies have suggested the addition of autologous PRP to the lipoaspirate. PRP is obtained from peripheral vein blood placed in sodium citrate anticoagulant and centrifugated (46,47). This process separates the red blood cells from the plasma, containing most (>90%) platelets. The obtained PRP is a natural reservoir of growth factors stimulating tissue repair and regeneration. It can be directly added to the lipoaspirate without any preconditioning, offering the advantage of a simple and cost-effective method. Animal models (48-51) first showed that the fat graft survival rate was significantly increased by adding PRP. Clinical studies start confirming these results: Gentile et al. (52) showed 69% graft retention when combining fat with PRP, which was significantly higher than fat grafting alone (39%) in their series of 50 patients. Several studies have been conducted on face treatment using fat transfer, showing similarly positive results (53-55). Finally, using endothelial cells has shown excellent results in preclinical models for enhancing fat grafting survivability (56).
A second approach uses the stromal vascular fraction (SVF) and adipose-derived mesenchymal stromal (or stem) cells (Figure 3). The initial isolated fat tissue is composed of adipocytes and stromal vascular fraction cells, which act as a cellular matrix and are composed of adipose stromal cells, preadipocytes, fibroblasts, vascular endothelial cells, muscle cells, pericytes, and a variety of immune cells (57). Most fat grafts’ regenerative capacity is attributed to adipose-derived mesenchymal stem cells (AD-MSCs) in SVF (58). Several studies in the past decade have shown that stromal vascular fraction cells and adipose stem cells improve fat graft survival through both angiogenic properties and growth factor production (59-63). Recent biotechnological developments offer the possibility to engineer the fat and enrich it with SVF (61). Several protocols exist aiming to isolate this fraction (62,63). The standard enzymatic method consists of digesting the lipoaspirate by a collagenase, followed by centrifugation to obtain a pellet at the bottom of the tube, containing the high-density adipose tissue (59). Other methods include spectroscopy or mechanical nano-fat techniques (oily liquid obtained after several steps of emulsification and filtration) (63-66). In vitro analyses emphasized filtration as a highly efficient mechanical technique for preserving the cell structures, while in vivo evidence seems to provide more promising results with enzymatic digestion (63,65). A prospective study compared the outcomes between several commercialized systems and found significant improvement with both enzymatic and mechanical-based systems (66). Enrichment with SVF, therefore, improves fat grafting by providing a bioengineered scaffold through cellular suspensions. Some studies have also suggested that SVF can lead to endothelial cell growth both in vitro and in vivo, enhancing the revascularization of fat grafts (67). However, to date, no formal proof of the differentiation of AD-MSCs into endothelial cells has been described, and endothelial progenitors contained in the SVF could be a likely explanation. One group more specifically tested human mononuclear cells containing endothelial progenitor cells and demonstrated increased fat graft survival in a rodent model (68). Overall, a safer approach is to consider the angiogenic potential of SVF cells as a whole through endothelial progenitors, AD-MSCs paracrine angiogenic activity, and potential AD-MSCs differentiation into endothelial cells. Further studies could focus more specifically on using human endothelial cells to enhance fat grafting survivability.
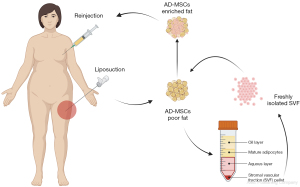
Clinical results with these techniques have already shown improved soft tissue volume maintenance and skin quality for scar repair and wound healing (64). In breast cancer reconstruction, SVF-enhanced autologous fat grafts present significantly better postoperative outcomes and contour maintenance at 1-year follow-up (58). However, the potential for an increased risk of breast cancer after injecting stem cells lacked adequate evidence for a long time. Mazur et al. (69) compared patients undergoing breast augmentation using SVF-enhanced lipofilling following a mastectomy or lumpectomy with patients not undergoing reconstruction. They matched both groups and showed no statistically significant difference in cancer recurrence after three years. The recurrence rate of the SVF group was even slightly lower (3.7% versus 4.13%) than the control group. Similarly, Calabrese et al. (70) prospectively compared conventional fat transfer and SVF-enhanced fat transfer in breast cancer patients. They had 40 to 60 patients in each of the fat grafting, SVF-enhanced fat grafting, and no reconstruction (control) groups, and the follow-up was 5 years. Again, they showed no difference between groups and a slightly lower recurrence in the SVF-enhanced group than in conventional fat grafting. Bielli et al. greatly reviewed the literature on this topic (71), highlighting the safety of adipose-derived stromal cell (ASC) and SVF enrichment of fat grafting with no significant change in the prognosis. They also performed a systematic review confirming these findings by emphasizing autologous fat grafting safety with no increased local-regional recurrences and no decreased disease-free survival (72). They also brought to light the poor amount of Level 1 evidence studies, such as international multicenter randomized studies, which should be promoted. In addition, it has been shown that magnetic resonance imaging allows for post-lipofilling breast monitoring, with experienced radiologists being able to distinguish malignant alterations from fat-related changes if patients are checked regularly (73).
An innovative study conducted in Italy in 2012 (74) compared 3D volume and contour maintenance following SVF- and PRP-enhanced fat grafting, with classic fat transfer as a control. Interestingly, they showed a similar enhancement of fat survival with 63% and 69% of volume maintenance after 1 year with SVF and PRP, respectively.
Another angle to approach fat grafting survivability enhancement is towards using bio-protectant molecules. William Austen Jr and his team published in 2011 impressive results in a preclinical study while testing several poloxamer molecules on fat grafting (75). Poloxamers are triblock copolymers composed of polyoxyethylene (PEO) and polyoxypropylene (PPO) segments in different proportions. One of the poloxamers, specifically poloxamer 188 (P188), is considered highly promising for plastic surgery due to its ability to repair cell membranes and other beneficial biological characteristics (76-78). The initial hypothesis was that it is possible to improve cell survival by repairing membrane damage created during fat harvesting, reducing apoptosis in the days following grafting. It has been previously demonstrated that volume maintenance was improved by gentle manipulation of the fat (79). This team from the Massachusetts General Hospital showed that grafts treated with P188 exhibited a 50 percent decrease in apoptosis compared to the saline-treated controls and achieved a remarkable 72 percent weight-based survival after 6 weeks. These results are confirmed by another study (80), assessing DNA contents using PicoGreen analyses, which showed higher fat survivability with P188. These findings led to the development of fat-processing systems (Vitality, SIENTRA, Irvine, CA, USA), with ongoing multicenter studies to clinically confirm these excellent results. Their preliminary results in the 13 enrolled centers show more than 86% retention after 6 months (81) needs to be confirmed at further time points. Another team (82,83) used this P188-based system to assess fat concentration and showed higher adipose volume (89%) when compared with other systems using a conventional Ringer rinsing solution. This approach could lead to better results in volume retention following fat grafting, eventually avoiding implant-based reconstructions and their complications.
It is worth noting that the legislative regulations need to evolve accordingly to some of these advances. Raposio and Ciliberti (83) highlighted that, according to the European Medicines Agency (EMA), ASCs are not classified as advanced therapy medicinal products if they have not undergone substantial manipulation, and their mode of action is considered homologous to the donor fat tissue, contributing to tissue renewal and turnover in the subcutaneous tissue. In contrast, substantial manipulations, such as collagenase digestion and cell culturing, are not allowed for ASCs to maintain their non-advanced therapy status. To date, only non-manipulated ASCs can be transplanted into the same anatomical or histological environment to be considered homologous. It is clear that ongoing studies need to show strong evidence before leading to changes in these regulations.
Building breast volume and shape through bio-printing
Breast volume and shape restoration is a critical stage in the breast reconstruction process. Traditionally, this is accomplished using silicone implants or autologous tissue transfers, either free or pedicled flaps, or fat grafting. Implant-based reconstructions offer several advantages, including immediate results, relatively simpler procedures, and shorter recovery times for patients; autologous flaps provide the opportunity to reconstruct both the soft tissue envelope and the breast volume, often resulting in superior long-term outcomes compared to silicone implants (84,85). However, both techniques have inherent drawbacks, which create a need to develop new perspectives in breast reconstruction. Fat grafting can recreate breast mechanical properties that closely resemble those of a natural breast. Nevertheless, there are instances where fat grafting alone may be insufficient, leading to inadequate volume or inadequate projection of the reconstructed breast mound due to the absence of three-dimensional structural support and resorption (86). Tissue engineering teams have tried to address this issue. This led to the development of 3D-printed scaffolds that could potentially provide the needed structural support and avoid resorption.
Tissue printing is an old concept. The first 3D patent was granted in 1986, and the first description of bioprinting was in 2003 (87). Later, in 2011, Melchels et al. introduced 3D printing in breast reconstruction (88). These groundbreaking works paved the way for developing 3D bio-printed implants in breast surgery. This innovative approach holds promise in combining the benefits of both implant-based reconstruction and autologous reconstruction. An advantage of 3D printing is the potential to tailor-print a resorbable implant to match the size of a defect, in the particular case of breast-conservative tumor removal. Magnetic resonance imaging can indeed precisely match the implant to the expected defect before surgery, enabling genuinely personalized manufacturing (89). Additionally, the versatility of materials available, including commonly used ones such as polycaprolactone (PCL), polylactic acid (PLA), and poly(lactic-co-glycolic acid) (PLGA), along with the potential to incorporate cell-loaded bio-inks, opens up limitless possibilities for implant creation.
The scaffold-guided approach aims to implant a scaffold that can effectively guide patient tissue ingrowth (Figure 4). While this approach shows promise, first-generation implants have primarily shown the formation of fibrous tissue with an unnatural hard texture (90,91). However, creating a flexible structure and adding adipose-derived stem cells (ADSCs) to the construct allowed for enhancing this process and improving the outcomes (92). Moreover, optimizing the placement of cells within the scaffold can further improve engraftment and leverage the structural possibilities offered by 3D printing, combined with the tissue regeneration properties of fat grafting. Chhaya et al. demonstrated that delayed fat grafting two weeks post-implantation promotes angiogenesis and adipose tissue regeneration, allowing for the generation of large volumes of adipose tissue in a preclinical model (93).
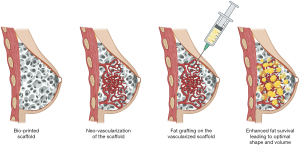
Mohseni et al. refined this technique. They developed a modular construct comprising an external layer for structural support and internal content that enhances fat grafting (94). However, two main challenges are associated with this approach. The first is achieving proper scaffold vascularization to ensure successful fat engraftment. The second challenge involves effectively managing the scaffold’s resorption phase and addressing the construct’s transient properties, which should mimic native breast tissue (95,96). Alternative methods involving bio-regenerative fillers composed of collagen have been proposed as potential solutions (97). Bio regenerative filling may serve as an alternative to a complete scaffold, particularly in oncologic breast conservative surgery cases.
The concept of a resorbable 3D printed structure has also let to the development of tissue engineering chambers (TECs). Morrison et al. first clinically tested this concept in Australia in 2016 (98). Their TEC involved an acrylic, perforated dome-shaped chamber that creates a dead space in which a pedicled fat flap is placed. The rest of the cavity is progressively filled with a seroma, ultimately leading to flap hypertrophy, providing volume. One major drawback of this method is the unnatural appearance of the breast due to the non-resorbable implant that requires a follow-up procedure for removal. More recently, Lattice Medical (Loos, France) improved this aspect by developing a bio-absorbable implant [Mat(T)isse] that fully degrades within 12 months, allowing for a one-stage surgical protocol (99-101). This implant is currently undergoing a clinical trial in Europe and has so far shown promising results. Another brand (Bellaseno GmbH, Leipzig, Germany) is working on a similar project and has manufactured a resorbable scaffold (Senella PCL Breast Scaffold) made of PCL that degrades in 24 to 36 months. This scaffolding is intended for both breast augmentation and reconstruction (102).
The concept of 4D printing introduces a new level of complexity and opens exciting possibilities in manufacturing implants. With 4D printing, implants can have characteristics that evolve over time, adapting to the patient’s body’s mechanical, physical, or enzymatic constraints (103). This advancement allows for better control over the resorption of biomaterials, transforming it into an opportunity to shape and modify the implant’s properties as needed. While still in its early stages, 4D printing holds immense potential for expanding the range of possibilities in implant technology. Furthermore, 4D printing enables the inclusion of drugs on the scaffold, facilitating local delivery for long-lasting and controlled diffusion (104). This feature can be used to achieve antitumoral effects (105-108) and even prevent post-operative complications such as thrombosis or infection (109).
Several authors have conducted research on animal models to explore the possibility of incorporating cells directly into 3D-printed breast structures. For example, human umbilical vein endothelial cells (HUVECs) (110) and human adipose-derived stromal cells (hADSCs) (111) have been investigated. Using bio-inks composed of hydrogels loaded with cells appears to be a promising approach, as it has demonstrated high cell survival rates (112). In a study by Sokol et al., primary human breast cells were successfully isolated and incorporated into a hydrogel, resulting in the regeneration of functional breast tissue (113). This tissue exhibited hormone responsiveness and was able to generate lipid droplets. Integrating cells directly into the bionic structure offers several advantages, such as accelerating adipose tissue regeneration and enhancing the adipose and vascular content of the reconstructed breast. However, current approaches have been restricted to animal testing. This limitation is partly due to potential concerns regarding the inclusion of cells in the bionic structure, as it may increase the risk of cancer recurrence. Before clinical applications can be implemented, it is crucial to assess the oncological safety of this approach, particularly for oncologic reconstruction (114).
NAC engineered reconstructions
Nipple reconstruction is a crucial component of breast reconstruction, as it significantly influences patient satisfaction with the overall outcome (115). The natural appearance of the NAC is of utmost importance (116), with factors such as position, color, projection, and texture playing a significant role. While autologous techniques are commonly used for nipple reconstruction, they may not always meet patients’ specific needs in the long term (117-124). Surgeons have attempted various approaches to restore the NAC’s natural appearance, including 3D tattoos, autologous skin or mucosal grafts, and local flaps. However, the absence of a consensus on a gold standard technique among the multitude of autologous approaches underscores the necessity for further advancements in the field. Thus, a growing body of literature has focused on innovative strategies for reconstructing the NAC, offering promising advancements in achieving a natural and aesthetically pleasing outcome. This summary outlines the key trends in this field.
Local flap nipple reconstruction is usually associated with a cartilage graft, including autologous (125), or banked costal cartilage (126), in order to improve the structural support of the nipple. However, these grafts often come with drawbacks such as donor site morbidity (if autologous) and resorption, which can lead to long-term nipple projection loss. To address these issues, biomaterials have been explored as potential solutions. Acellular dermal matrix (127-129), calcium hydroxyapatite (130,131), lyophilized allogenic costal cartilage (132), and biological collagen implants (133,134) have garnered significant interest as potential options in this regard. However, their cost and lack of long-term support are often perceived as limitations to their utilization. Injectables, increasingly favored by surgeons for their convenience in outpatient settings, have also been gaining popularity. Among them, hyaluronic acid (135,136), and biomaterials derived from bovine collagen (137) present exciting alternatives to retro-areolar fat grafting.
Synthetic materials with adequate biocompatibility, such as silicone and polytetrafluoroethylene (PTFE) (138), have been explored for ready-to-use and long-lasting internal NAC prosthetics. Jankau et al. reported high complication rates with frequent (if not systematic) local necrosis and implant extrusion after experimenting with implantable silicone rods (139). This may be attributed to the heightened pressure exerted by the silicone rod on the flap used for breast reconstruction. FixNip NRI (FixNip LTD, Caesarea, Israel) is a silicone flexible Nipple reconstruction implant (NRI) approved for sale in Europe and currently under FDA review. Its internal Nitinol frame allows for a flexible yet reliable and long-lasting reconstruction of the NAC in a surgical-friendly manner (140). Although this implant also carries the risk of infection and extrusion, it has not proven to be problematic.
Another notable trend is the use of 3D printing technology. Several techniques already discussed in the previous section (3.3) can be applied to NAC engineering and imply either printing a resorbable scaffold or directly bio-printing cell-containing inks. The synergistic blend of scalability and production efficiency, combined with the inherent capacity to retain flexibility for customization, confers significant advantages when considering industrial production. Vernice et al. described a 3D-printed PLA scaffold with an ovine decellularised infill (141), while Samadi et al. opted for a costal cartilage fill (142). Similarly, Dong et al. report using a 3D printed poly-4-hydroxybutyrate (P4HB) that is a fully resorbable FDA-cleared biomaterial to construct scaffolds filled with processed costal cartilage (143). With these techniques, nipple projection was maintained over time with natural biomechanics properties. In cell-containing bio-inks techniques, ADSCs offer the hypothetical possibility of combining the structural benefits of the 3D printed scaffold with the regenerative properties of the ADSCs. This theoretical advantage needs to be weighed against the inherent limitation of these constructs, as they lack cellular differentiations necessary for optimal structural function (144). An alternative approach could be using bio incubators to facilitate cell maturation and the subsequent implantation of differentiated tissue. However, regardless of the technique employed, autologous cell seeding into the scaffold proves to be a costly solution, and the resulting implant is not readily deployable, thereby limiting the practicality of this approach, according to certain authors.
With the advent of decellularization bioengineering techniques (143,144), exciting advancements have been made in developing NAC scaffolds for tissue regeneration. These innovative technologies, such as ADMs, have been proven safe and are commercially available, finding widespread clinical applications. Numerous studies have demonstrated that acellular nipple scaffolds (ANS) are free from cellular antigens and effectively cleared of DNA (145), using the same threshold of 50 ng/mg of tissue, thereby minimizing the risk of rejection by the recipient’s immune system. Moreover, these scaffolds maintain the integrity and bioactivity of the extracellular matrix, enabling successful reseeding with cells (146,147). They have also shown the capacity to support host-mediated re-cellularization with the growth of epithelial cells, neo-vasculature, and even nerve ingrowth (148). While proof of concept has been achieved using non-human-primate-derived ANS (149), concerns regarding ethics, cost, and limited availability have prompted our team to explore porcine-originated scaffolds with promising results (Figure 5), either as a preclinical model or as a genuine source of ANS (145). Re-cellularization with autologous cells can further enhance the outcomes of bioengineered nipple grafts. This approach allows for better control over scaffold contracture and facilitates quicker integration in the recipient, thereby achieving a fully tolerated and successful bioengineered nipple graft. Our team is actively investigating this exciting avenue to eventually achieve a perfect match between the scaffold and the recipient.
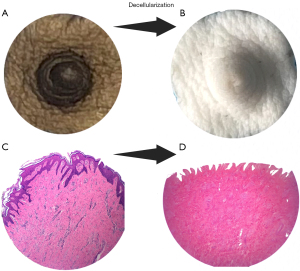
Strengths and limitations
Our article demonstrates strength in providing a broad and accessible insight into the current landscape and future prospects of tissue engineering applied to breast reconstruction. It discusses a range of techniques, from stem cells and scaffolds to 3D bioprinting, using a comprehensive, yet understandable style. However, the inherent limitations of the narrative review format are apparent, including potential bias due to its non-systematic nature, and the absence of in-depth discussion of the different topics. Additionally, the speculations on future advancements, although intriguing, remain mostly hypothetical due to the rapidly evolving nature of this field.
Conclusions
Tissue and Bio- engineering represent a promising approach for breast reconstruction. These innovative approaches can eventually address multiple steps of the complex process of breast reconstruction. An asymmetrical evolution is certain, with some processes already in use while others are in the early stages of development. Combination with autologous techniques can help reach the expected results, which are a complete, precise and stable restoration of the reconstructed breast’s appearance, as well as its functional impact as a part of women’s femininity.
Acknowledgments
Funding: This work was supported by
Footnote
Reporting Checklist: The authors have completed the Narrative Review reporting checklist. Available at https://atm.amegroups.com/article/view/10.21037/atm-23-1724/rc
Peer Review File: Available at https://atm.amegroups.com/article/view/10.21037/atm-23-1724/prf
Conflicts of Interest: All authors have completed the ICMJE uniform disclosure form (available at https://atm.amegroups.com/article/view/10.21037/atm-23-1724/coif). A.G.L. serves as an unpaid editorial board member of Annals of Translational Medicine from December 2022 to November 2024. The other authors have no conflicts of interest to declare.
Ethical Statement: The authors are accountable for all aspects of the work in ensuring that questions related to the accuracy or integrity of any part of the work are appropriately investigated and resolved.
Open Access Statement: This is an Open Access article distributed in accordance with the Creative Commons Attribution-NonCommercial-NoDerivs 4.0 International License (CC BY-NC-ND 4.0), which permits the non-commercial replication and distribution of the article with the strict proviso that no changes or edits are made and the original work is properly cited (including links to both the formal publication through the relevant DOI and the license). See: https://creativecommons.org/licenses/by-nc-nd/4.0/.
References
- Giaquinto AN, Sung H, Miller KD, et al. Breast Cancer Statistics, 2022. CA Cancer J Clin 2022;72:524-41. [Crossref] [PubMed]
- Jonczyk MM, Jean J, Graham R, et al. Surgical trends in breast cancer: a rise in novel operative treatment options over a 12 year analysis. Breast Cancer Res Treat 2019;173:267-74. [Crossref] [PubMed]
- Engels PE, Thuerlimann AC, Schulz SN, et al. Breast reconstruction after mastectomy: necessity, evolution, techniques and research goals. Rev Med Suisse 2023;19:1180-5. [Crossref] [PubMed]
- Lin AM, Lorenzi R, Van Der Hulst JE, et al. A Decade of Nipple-Sparing Mastectomy: Lessons Learned in 3035 Immediate Implant-based Breast Reconstructions. Plast Reconstr Surg 2023; Epub ahead of print. [Crossref]
- Galimberti V, Vicini E, Corso G, et al. Nipple-sparing and skin-sparing mastectomy: Review of aims, oncological safety and contraindications. Breast 2017;34:S82-4. [Crossref] [PubMed]
- Malekpour M, Malekpour F, Wang HT. Breast reconstruction: Review of current autologous and implant-based techniques and long-term oncologic outcome. World J Clin Cases 2023;11:2201-12. [Crossref] [PubMed]
- Gronovich Y, Maisel-Lotan A. An Elegant Method for Removing Breast Implants - a Novel Surgical Device. Plast Reconstr Surg 2023; Epub ahead of print. [Crossref]
- Speck NE, Grufman V, Farhadi J. Trends and Innovations in Autologous Breast Reconstruction. Arch Plast Surg 2023;50:240-7. [Crossref] [PubMed]
- Momeni A, Becker A, Torio-Padron N, et al. Nipple reconstruction: evidence-based trials in the plastic surgical literature. Aesthetic Plast Surg 2008;32:18-20. [Crossref] [PubMed]
- Vendemia N, Mesbahi AN, McCarthy CM, et al. Nipple areola reconstruction. Cancer J 2008;14:253-7. [Crossref] [PubMed]
- Langer R, Vacanti JP. Tissue engineering. Science 1993;260:920-6. [Crossref] [PubMed]
- Peretti GM, Zaporojan V, Spangenberg KM, et al. Cell-based bonding of articular cartilage: An extended study. J Biomed Mater Res A 2003;64:517-24. [Crossref] [PubMed]
- Roy R, Kohles SS, Zaporojan V, et al. Analysis of bending behavior of native and engineered auricular and costal cartilage. J Biomed Mater Res A 2004;68:597-602. [Crossref] [PubMed]
- Baethge C, Goldbeck-Wood S, Mertens S. SANRA-a scale for the quality assessment of narrative review articles. Res Integr Peer Rev 2019;4:5. [Crossref] [PubMed]
- Crapo PM, Gilbert TW, Badylak SF. An overview of tissue and whole organ decellularization processes. Biomaterials 2011;32:3233-43. [Crossref] [PubMed]
- Hassan AM, Asaad M, Brook DS, et al. Outcomes of Abdominal Wall Reconstruction with a Bovine versus a Porcine Acellular Dermal Matrix: A Propensity Score-Matched Analysis. Plast Reconstr Surg 2023;152:872-81. [Crossref] [PubMed]
- Ilahi ON, Velmahos G, Janis JE, et al. Prospective, multicenter study of antimicrobial-coated, noncrosslinked, acellular porcine dermal matrix (XenMatrix™ AB Surgical Graft) for hernia repair in all centers for disease control and prevention wound classes: 24-month follow-up cohort. Ann Med Surg (Lond) 2023;85:1571-7. [Crossref] [PubMed]
- Gierek M, Łabuś W, Kitala D, et al. Human Acellular Dermal Matrix in Reconstructive Surgery-A Review. Biomedicines 2022;10:2870. [Crossref] [PubMed]
- Capito AE, Tholpady SS, Agrawal H, et al. Evaluation of host tissue integration, revascularization, and cellular infiltration within various dermal substrates. Ann Plast Surg 2012;68:495-500. [Crossref] [PubMed]
- Scarano A, Barros RR, Iezzi G, et al. Acellular dermal matrix graft for gingival augmentation: a preliminary clinical, histologic, and ultrastructural evaluation. J Periodontol 2009;80:253-9. [Crossref] [PubMed]
- Taufique ZM, Bhatt N, Zagzag D, et al. Revascularization of AlloDerm Used during Endoscopic Skull Base Surgery. J Neurol Surg B Skull Base 2019;80:46-50. [Crossref] [PubMed]
- Pires G, Marquez JL, Memmott S, et al. An Evaluation of Early Complications after Prepectoral Tissue Expander Placement in First-Stage Breast Reconstruction with and without Acellular Dermal Matrix. Plast Reconstr Surg 2023; Epub ahead of print. [Crossref]
- Headon H, Kasem A, Mokbel K. Capsular Contracture after Breast Augmentation: An Update for Clinical Practice. Arch Plast Surg 2015;42:532-43. [Crossref] [PubMed]
- Samaha Y, Chen J, Ray EC. ADMs and synthetic meshes improve implant-based breast reconstruction aesthetics, but at what cost? J Plast Reconstr Aesthet Surg 2023;80:178-81. [Crossref] [PubMed]
- Podsednik Gardner A, Nunez A, De la Garza M. Red Breast Syndrome and Acellular Dermal Matrix. Plast Reconstr Surg Glob Open 2023;11:e5062. [Crossref] [PubMed]
- Danino MA, El Khatib AM, Doucet O, et al. Preliminary Results Supporting the Bacterial Hypothesis in Red Breast Syndrome following Postmastectomy Acellular Dermal Matrix- and Implant-Based Reconstructions. Plast Reconstr Surg 2019;144:988e-92e. [Crossref] [PubMed]
- Myckatyn TM. Discussion: Preliminary Results Supporting the Bacterial Hypothesis in Red Breast Syndrome following Postmastectomy Acellular Dermal Matrix- and Implant-Based Reconstructions. Plast Reconstr Surg 2019;144:993e-4e. [Crossref] [PubMed]
- Nguyen TC, Brown AM, Kulber DA, et al. The Role of Endotoxin in Sterile Inflammation After Implanted Acellular Dermal Matrix: Red Breast Syndrome Explained? Aesthet Surg J 2020;40:392-9. [Crossref] [PubMed]
- Gentile P, Bernini M, Orzalesi L, et al. Titanium-coated polypropylene mesh as innovative bioactive material in conservatives mastectomies and pre-pectoral breast reconstruction. Bioact Mater 2021;6:4640-53. [Crossref] [PubMed]
- Graziano FD, Plotsker EL, Rubenstein RN, et al. National Trends in Acellular Dermal Matrix Utilization in Immediate Breast Reconstruction. Plast Reconstr Surg 2023; Epub ahead of print. [Crossref]
- Ellsworth WA 4th, Hammer J, Luo L, et al. Acellular Dermal Matrices in Breast Reconstruction: CARE Trial 5-Year Outcomes Data for More Than 9500 Patients. Plast Reconstr Surg Glob Open 2022;10:e4258. [Crossref] [PubMed]
- Forsberg CG, Kelly DA, Wood BC, et al. Aesthetic outcomes of acellular dermal matrix in tissue expander/implant-based breast reconstruction. Ann Plast Surg 2014;72:S116-20. [Crossref] [PubMed]
- Ibrahim AM, Koolen PG, Ganor O, et al. Does acellular dermal matrix really improve aesthetic outcome in tissue expander/implant-based breast reconstruction? Aesthetic Plast Surg 2015;39:359-68. [Crossref] [PubMed]
- Wilson RL, Kirwan CC, O'Donoghue JM, et al. BROWSE: A multicentre comparison of nine year outcomes in acellular dermal matrix based and complete submuscular implant-based immediate breast reconstruction-aesthetics, capsular contracture and patient reported outcomes. Eur J Surg Oncol 2022;48:73-81. [Crossref] [PubMed]
- Pusic AL, Klassen AF, Scott AM, et al. Development of a new patient-reported outcome measure for breast surgery: the BREAST-Q. Plast Reconstr Surg 2009;124:345-53. [Crossref] [PubMed]
- Tasoulis MK, Teoh V, Khan A, et al. Acellular dermal matrices as an adjunct to implant breast reconstruction: Analysis of outcomes and complications. Eur J Surg Oncol 2020;46:511-5. [Crossref] [PubMed]
- de Blacam C, Momoh AO, Colakoglu S, et al. Cost analysis of implant-based breast reconstruction with acellular dermal matrix. Ann Plast Surg 2012;69:516-20. [Crossref] [PubMed]
- Bank J, Phillips NA, Park JE, et al. Economic analysis and review of the literature on implant-based breast reconstruction with and without the use of the acellular dermal matrix. Aesthetic Plast Surg 2013;37:1194-201. [Crossref] [PubMed]
- Krishnan NM, Chatterjee A, Rosenkranz KM, et al. The cost effectiveness of acellular dermal matrix in expander-implant immediate breast reconstruction. J Plast Reconstr Aesthet Surg 2014;67:468-76. [Crossref] [PubMed]
- Strong AL, Cederna PS, Rubin JP, et al. The Current State of Fat Grafting: A Review of Harvesting, Processing, and Injection Techniques. Plast Reconstr Surg 2015;136:897-912. [Crossref] [PubMed]
- Gentile P. Tuberous Breast, Deformities, and Asymmetries: A Retrospective Analysis Comparing Fat Grafting Versus Mastopexy and Breast Implants. Aesthetic Plast Surg 2023;47:1683-94. [Crossref] [PubMed]
- Araco A, Gravante G, Araco F, et al. Breast asymmetries: a brief review and our experience. Aesthetic Plast Surg 2006;30:309-19. [Crossref] [PubMed]
- Gentile P. Breast Silicone Gel Implants versus Autologous Fat Grafting: Biomaterials and Bioactive Materials in Comparison. J Clin Med 2021;10:3310. [Crossref] [PubMed]
- Al Sufyani MA, Al Hargan AH, Al Shammari NA, et al. Autologous Fat Transfer for Breast Augmentation: A Review. Dermatol Surg 2016;42:1235-42. [Crossref] [PubMed]
- Erdim M, Tezel E, Numanoglu A, et al. The effects of the size of liposuction cannula on adipocyte survival and the optimum temperature for fat graft storage: an experimental study. J Plast Reconstr Aesthet Surg 2009;62:1210-4. [Crossref] [PubMed]
- Wu M, Karvar M, Liu Q, et al. Comparison of Conventional and Platelet-Rich Plasma-Assisted Fat Grafting: A Systematic Review and Meta-analysis. J Plast Reconstr Aesthet Surg 2021;74:2821-30. [Crossref] [PubMed]
- Nilforoushzadeh MA, Heidari-Kharaji M, Alavi S, et al. Transplantation of autologous fat, stromal vascular fraction (SVF) cell, and platelet-rich plasma (PRP) for cell therapy of atrophic acne scars: Clinical evaluation and biometric assessment. J Cosmet Dermatol 2022;21:2089-98. [Crossref] [PubMed]
- Lei X, Liu H, Pang M, et al. Effects of Platelet-Rich Plasma on Fat and Nanofat Survival: An Experimental Study on Mice. Aesthetic Plast Surg 2019;43:1085-94. [Crossref] [PubMed]
- Nakamura S, Ishihara M, Takikawa M, et al. Platelet-rich plasma (PRP) promotes survival of fat-grafts in rats. Ann Plast Surg 2010;65:101-6. [Crossref] [PubMed]
- Xiong BJ, Tan QW, Chen YJ, et al. The Effects of Platelet-Rich Plasma and Adipose-Derived Stem Cells on Neovascularization and Fat Graft Survival. Aesthetic Plast Surg 2018;42:1-8. [Crossref] [PubMed]
- Zheng H, Yu Z, Deng M, et al. Fat extract improves fat graft survival via proangiogenic, anti-apoptotic and pro-proliferative activities. Stem Cell Res Ther 2019;10:174. [Crossref] [PubMed]
- Gentile P, Di Pasquali C, Bocchini I, et al. Breast reconstruction with autologous fat graft mixed with platelet-rich plasma. Surg Innov 2013;20:370-6. [Crossref] [PubMed]
- Alkerdi K, Alsabek L, Alkhouli M, et al. Evaluation of the effect of injectable platelet-rich fibrin (I-PRF) in reducing the resorption of fat graft during facial lipostructure: A randomized clinical trial. Dent Med Probl 2022;59:131-6. [Crossref] [PubMed]
- Zhang Z, Qiu L, Cui D, et al. Use of platelet-rich fibrin in fat grafts during facial lipostructure. Front Surg 2022;9:923342. [Crossref] [PubMed]
- Zhang ZX, Qiu LH, Shi N, et al. Platelet-Rich Fibrin in Fat Grafts for Facial Lipofilling: A Randomized, Controlled Split-Face Clinical Trial. Front Surg 2022;9:793439. [Crossref] [PubMed]
- Debuc B, Gendron N, Cras A, et al. Improving Autologous Fat Grafting in Regenerative Surgery through Stem Cell-Assisted Lipotransfer. Stem Cell Rev Rep 2023;19:1726-54. [Crossref] [PubMed]
- Bourin P, Bunnell BA, Casteilla L, et al. Stromal cells from the adipose tissue-derived stromal vascular fraction and culture expanded adipose tissue-derived stromal/stem cells: a joint statement of the International Federation for Adipose Therapeutics and Science (IFATS) and the International Society for Cellular Therapy (ISCT). Cytotherapy 2013;15:641-8. [Crossref] [PubMed]
- Gentile P, Sterodimas A, Calabrese C, et al. Systematic review: Advances of fat tissue engineering as bioactive scaffold, bioactive material, and source for adipose-derived mesenchymal stem cells in wound and scar treatment. Stem Cell Res Ther 2021;12:318. [Crossref] [PubMed]
- Condé-Green A, Wu I, Graham I, et al. Comparison of 3 techniques of fat grafting and cell-supplemented lipotransfer in athymic rats: a pilot study. Aesthet Surg J 2013;33:713-21. [Crossref] [PubMed]
- Philips BJ, Grahovac TL, Valentin JE, et al. Prevalence of endogenous CD34+ adipose stem cells predicts human fat graft retention in a xenograft model. Plast Reconstr Surg 2013;132:845-58. [Crossref] [PubMed]
- Gentile P, Casella D, Palma E, et al. Engineered Fat Graft Enhanced with Adipose-Derived Stromal Vascular Fraction Cells for Regenerative Medicine: Clinical, Histological and Instrumental Evaluation in Breast Reconstruction. J Clin Med 2019;8:504. [Crossref] [PubMed]
- Gentile P, Calabrese C, De Angelis B, et al. Impact of the Different Preparation Methods to Obtain Human Adipose-Derived Stromal Vascular Fraction Cells (AD-SVFs) and Human Adipose-Derived Mesenchymal Stem Cells (AD-MSCs): Enzymatic Digestion Versus Mechanical Centrifugation. Int J Mol Sci 2019;20:5471. [Crossref] [PubMed]
- Gentile P, Cervelli V, De Fazio D, et al. Mechanical and Enzymatic Digestion of Autologous Fat Grafting (A-FG): Fat Volume Maintenance and AD-SVFs Amount in Comparison. Aesthetic Plast Surg 2023;47:2051-62. [Crossref] [PubMed]
- Tonnard P, Verpaele A, Peeters G, et al. Nanofat grafting: basic research and clinical applications. Plast Reconstr Surg 2013;132:1017-26. [Crossref] [PubMed]
- Girard P, Dulong J, Duisit J, et al. Modified nanofat grafting: Stromal vascular fraction simple and efficient mechanical isolation technique and perspectives in clinical recellularization applications. Front Bioeng Biotechnol 2022;10:895735. [Crossref] [PubMed]
- Gentile P, Scioli MG, Orlandi A, et al. Breast Reconstruction with Enhanced Stromal Vascular Fraction Fat Grafting: What Is the Best Method? Plast Reconstr Surg Glob Open 2015;3:e406. [Crossref] [PubMed]
- Planat-Benard V, Silvestre JS, Cousin B, et al. Plasticity of human adipose lineage cells toward endothelial cells: physiological and therapeutic perspectives. Circulation 2004;109:656-63. [Crossref] [PubMed]
- Geeroms M, Fujimura S, Aiba E, et al. Quality and Quantity-Cultured Human Mononuclear Cells Improve Human Fat Graft Vascularization and Survival in an In Vivo Murine Experimental Model. Plast Reconstr Surg 2021;147:373-85. [Crossref] [PubMed]
- Mazur S, Zołocińska A, Siennicka K, et al. Safety of adipose-derived cell (stromal vascular fraction - SVF) augmentation for surgical breast reconstruction in cancer patients. Adv Clin Exp Med 2018;27:1085-90. [Crossref] [PubMed]
- Calabrese C, Kothari A, Badylak S, et al. Oncological safety of stromal vascular fraction enriched fat grafting in two-stage breast reconstruction after nipple sparing mastectomy: long-term results of a prospective study. Eur Rev Med Pharmacol Sci 2018;22:4768-77. [Crossref] [PubMed]
- Bielli A, Scioli MG, Gentile P, et al. Adipose Tissue-Derived Stem Cell Therapy for Post-Surgical Breast Reconstruction--More Light than Shadows. Adv Clin Exp Med 2015;24:545-8. [Crossref] [PubMed]
- Gentile P, Cervelli V. Systematic review: Oncological safety of reconstruction with fat grafting in breast cancer outcomes. J Plast Reconstr Aesthet Surg 2022;75:4160-8. [Crossref] [PubMed]
- Fiaschetti V, Pistolese CA, Fornari M, et al. Magnetic resonance imaging and ultrasound evaluation after breast autologous fat grafting combined with platelet-rich plasma. Plast Reconstr Surg 2013;132:498e-509e. [Crossref] [PubMed]
- Gentile P, Orlandi A, Scioli MG, et al. A comparative translational study: the combined use of enhanced stromal vascular fraction and platelet-rich plasma improves fat grafting maintenance in breast reconstruction. Stem Cells Transl Med 2012;1:341-51. [Crossref] [PubMed]
- Medina MA 3rd, Nguyen JT, Kirkham JC, et al. Polymer therapy: a novel treatment to improve fat graft viability. Plast Reconstr Surg 2011;127:2270-82. [Crossref] [PubMed]
- Sandor B, Marin M, Lapoumeroulie C, et al. Effects of Poloxamer 188 on red blood cell membrane properties in sickle cell anaemia. Br J Haematol 2016;173:145-9. [Crossref] [PubMed]
- Tang SE, Liao WI, Pao HP, et al. Poloxamer 188 Attenuates Ischemia-Reperfusion-Induced Lung Injury by Maintaining Cell Membrane Integrity and Inhibiting Multiple Signaling Pathways. Front Pharmacol 2021;12:650573. [Crossref] [PubMed]
- Zargari M, Meyer LJ, Riess ML, et al. P188 Therapy in In Vitro Models of Traumatic Brain Injury. Int J Mol Sci 2023;24:3334. [Crossref] [PubMed]
- Gentile P, De Angelis B, Di Pietro V, et al. Gentle Is Better: The Original "Gentle Technique" for Fat Placement in Breast Lipofilling. J Cutan Aesthet Surg 2018;11:120-6. [Crossref] [PubMed]
- Medina MA 3rd, Nguyen JT, McCormack MM, et al. A high-throughput model for fat graft assessment. Lasers Surg Med 2009;41:738-44. [Crossref] [PubMed]
- Sientra Inc. Preliminary Results From Multi-Center Volume Retention Study of Vitality™ with Auraclens™ Lipoaspirate Wash System Support Enhanced Viability Fat Transfer (press release, 2023). Available online: https://investors.sientra.com/news/news-details/2023/.
- An Y, Panayi AC, Mi B, et al. Comparative Analysis of Two Automated Fat-processing Systems. Plast Reconstr Surg Glob Open 2020;8:e2587. [Crossref] [PubMed]
- Raposio E, Ciliberti R. Clinical use of adipose-derived stem cells: European legislative issues. Ann Med Surg (Lond) 2017;24:61-4. [Crossref] [PubMed]
- Sgarzani R, Negosanti L, Morselli PG, et al. Patient Satisfaction and Quality of Life in DIEAP Flap versus Implant Breast Reconstruction. Surg Res Pract 2015;2015:405163. [Crossref] [PubMed]
- Levine SM, Lester ME, Fontenot B, et al. Perforator flap breast reconstruction after unsatisfactory implant reconstruction. Ann Plast Surg 2011;66:513-7. [Crossref] [PubMed]
- Leopardi D, Thavaneswaran P, Mutimer KL, et al. Autologous fat transfer for breast augmentation: a systematic review. ANZ J Surg 2014;84:225-30. [Crossref] [PubMed]
- Gross BC, Erkal JL, Lockwood SY, et al. Evaluation of 3D printing and its potential impact on biotechnology and the chemical sciences. Anal Chem 2014;86:3240-53. [Crossref] [PubMed]
- Melchels F, Wiggenhauser PS, Warne D, et al. CAD/CAM-assisted breast reconstruction. Biofabrication 2011;3:034114. [Crossref] [PubMed]
- Zhang J, Yao Q, Huang M, et al. Computer-assisted 3-dimensional printing technology for immediate breast reconstruction after breast-conserving surgery. Chinese Journal of Breast Disease 2018;1:12-6.
- Tsuji W, Inamoto T, Ito R, et al. Simple and longstanding adipose tissue engineering in rabbits. J Artif Organs 2013;16:110-4. [Crossref] [PubMed]
- Findlay MW, Dolderer JH, Trost N, et al. Tissue-engineered breast reconstruction: bridging the gap toward large-volume tissue engineering in humans. Plast Reconstr Surg 2011;128:1206-15. [Crossref] [PubMed]
- Tytgat L, Van Damme L, Ortega Arevalo MDP, et al. Extrusion-based 3D printing of photo-crosslinkable gelatin and κ-carrageenan hydrogel blends for adipose tissue regeneration. Int J Biol Macromol 2019;140:929-38. [Crossref] [PubMed]
- Chhaya MP, Balmayor ER, Hutmacher DW, et al. Transformation of Breast Reconstruction via Additive Biomanufacturing. Sci Rep 2016;6:28030. [Crossref] [PubMed]
- Mohseni M, Bas O, Castro NJ, et al. Additive biomanufacturing of scaffolds for breast reconstruction. Addit Manuf 2019;30:100845.
- Bao W, Cao L, Wei H, et al. Effect of 3D printed polycaprolactone scaffold with a bionic structure on the early stage of fat grafting. Mater Sci Eng C Mater Biol Appl 2021;123:111973. [Crossref] [PubMed]
- Zhou M, Hou J, Zhang G, et al. Tuning the mechanics of 3D-printed scaffolds by crystal lattice-like structural design for breast tissue engineering. Biofabrication 2019;12:015023. [Crossref] [PubMed]
- Puls TJ, Fisher CS, Cox A, et al. Regenerative tissue filler for breast conserving surgery and other soft tissue restoration and reconstruction needs. Sci Rep 2021;11:2711. [Crossref] [PubMed]
- Morrison WA, Marre D, Grinsell D, et al. Creation of a Large Adipose Tissue Construct in Humans Using a Tissue-engineering Chamber: A Step Forward in the Clinical Application of Soft Tissue Engineering. EBioMedicine 2016;6:238-45. [Crossref] [PubMed]
- Faglin P, Gradwohl M, Depoortere C, et al. Rationale for the design of 3D-printable bioresorbable tissue-engineering chambers to promote the growth of adipose tissue. Sci Rep 2020;10:11779. [Crossref] [PubMed]
- Gradwohl M, Chai F, Payen J, et al. Effects of Two Melt Extrusion Based Additive Manufacturing Technologies and Common Sterilization Methods on the Properties of a Medical Grade PLGA Copolymer. Polymers (Basel) 2021;13:572. [Crossref] [PubMed]
- Lattice Medical. Success of the first breast reconstruction surgery with Mattisse. (press release, 2022). Available online: https://www.lattice-medical.com/wp-content/uploads/2022/09/.
- BellaSeno GmbH. Positive Interim Data of BellaSeno’s Clinical Trial with Resorbable Breast and Chest Implants Reported at RACS 2023 (press release, 2023). Available online: https://www.bellaseno.com/news-and-events/.
- Tamay DG, Dursun Usal T, Alagoz AS, et al. 3D and 4D Printing of Polymers for Tissue Engineering Applications. Front Bioeng Biotechnol 2019;7:164. [Crossref] [PubMed]
- Moroni S, Casettari L, Lamprou DA. 3D and 4D Printing in the Fight against Breast Cancer. Biosensors (Basel) 2022;12:568. [Crossref] [PubMed]
- Dang HP, Hutmacher DW, Tran PA. The Use of 3D Printed Microporous-Strut Polycaprolactone Scaffolds for Targeted Local Delivery of Chemotherapeutic Agent for Breast Cancer Application. 7th International Conference on the Development of Biomedical Engineering in Vietnam (BME7); 2020; Singapore: Springer Singapore.
- Fan L, Mei Y, Gao C, et al. Three-dimensional printed 5-fluorouracil /UHMWPE scaffolds for the treatment of breast cancer. Bioprinting 2021;24:e00174.
- Qiao X, Yang Y, Huang R, et al. E-Jet 3D-Printed Scaffolds as Sustained Multi-Drug Delivery Vehicles in Breast Cancer Therapy. Pharm Res 2019;36:182. [Crossref] [PubMed]
- Yang Y, Qiao X, Huang R, et al. E-jet 3D printed drug delivery implants to inhibit growth and metastasis of orthotopic breast cancer. Biomaterials 2020;230:119618. [Crossref] [PubMed]
- Dang HP, Shabab T, Shafiee A, et al. 3D printed dual macro-, microscale porous network as a tissue engineering scaffold with drug delivering function. Biofabrication 2019;11:035014. [Crossref] [PubMed]
- Chhaya MP, Melchels FP, Holzapfel BM, et al. Sustained regeneration of high-volume adipose tissue for breast reconstruction using computer aided design and biomanufacturing. Biomaterials 2015;52:551-60. [Crossref] [PubMed]
- Rossi E, Guerrero J, Aprile P, et al. Decoration of RGD-mimetic porous scaffolds with engineered and devitalized extracellular matrix for adipose tissue regeneration. Acta Biomater 2018;73:154-66. [Crossref] [PubMed]
- Tytgat L, Van Damme L, Van Hoorick J, et al. Additive manufacturing of photo-crosslinked gelatin scaffolds for adipose tissue engineering. Acta Biomater 2019;94:340-50. [Crossref] [PubMed]
- Sokol ES, Miller DH, Breggia A, et al. Growth of human breast tissues from patient cells in 3D hydrogel scaffolds. Breast Cancer Res 2016;18:19. [Crossref] [PubMed]
- O'Halloran N, Courtney D, Kerin MJ, et al. Adipose-Derived Stem Cells in Novel Approaches to Breast Reconstruction: Their Suitability for Tissue Engineering and Oncological Safety. Breast Cancer (Auckl) 2017;11:1178223417726777. [Crossref] [PubMed]
- Egan KG, Cullom M, Nazir N, et al. Patient Satisfaction Increases with Nipple Reconstruction following Autologous Breast Reconstruction. Plast Reconstr Surg 2021;148:177e-84e. [Crossref] [PubMed]
- Pietruski P, Paskal W, Paskal AM, et al. Analysis of the Visual Perception of Female Breast Aesthetics and Symmetry: An Eye-Tracking Study. Plast Reconstr Surg 2019;144:1257-66. [Crossref] [PubMed]
- Few JW, Marcus JR, Casas LA, et al. Long-Term Predicatable Nipple Projection following Reconstruction. Plastic and Reconstructive Surgery 1999;104:1321-4. [Crossref] [PubMed]
- Zhong T, Antony A, Cordeiro P. Surgical outcomes and nipple projection using the modified skate flap for nipple-areolar reconstruction in a series of 422 implant reconstructions. Ann Plast Surg 2009;62:591-5. [Crossref] [PubMed]
- Guerra AB, Khoobehi K, Metzinger SE, et al. New technique for nipple areola reconstruction: arrow flap and rib cartilage graft for long-lasting nipple projection. Ann Plast Surg 2003;50:31-7. [Crossref] [PubMed]
- Sisti A, Grimaldi L, Tassinari J, et al. Nipple-areola complex reconstruction techniques: A literature review. Eur J Surg Oncol 2016;42:441-65. [Crossref] [PubMed]
- Butz DR, Kim EK, Song DH. C-Y Trilobed Flap for Improved Nipple-Areola Complex Reconstruction. Plast Reconstr Surg 2015;136:234-7. [Crossref] [PubMed]
- Yang JD, Ryu JY, Ryu DW, et al. Our Experiences in Nipple Reconstruction Using the Hammond flap. Arch Plast Surg 2014;41:550-5. [Crossref] [PubMed]
- Elizabeth Clark S, Turton E. The CC-V Flap: A Novel Technique for Augmenting a C-V Nipple Reconstruction Using a Free Dermal Graft. World J Plast Surg 2014;3:8-12.
- Farhadi J, Maksvytyte GK, Schaefer DJ, et al. Reconstruction of the nipple-areola complex: an update. J Plast Reconstr Aesthet Surg 2006;59:40-53. [Crossref] [PubMed]
- Guerid S, Boucher F, Mojallal A. Nipple reconstruction using rib cartilage strut in microsurgical reconstructed breast. Ann Chir Plast Esthet 2017;62:332-5. [Crossref] [PubMed]
- Mori H, Uemura N, Okazaki M. Nipple reconstruction with banked costal cartilage after vertical-type skin-sparing mastectomy and deep inferior epigastric artery perforator flap. Breast Cancer 2015;22:95-7. [Crossref] [PubMed]
- Chen WF, Barounis D, Kalimuthu R. A novel cost-saving approach to the use of acellular dermal matrix (AlloDerm) in postmastectomy breast and nipple reconstructions. Plast Reconstr Surg 2010;125:479-81. [Crossref] [PubMed]
- Garramone CE, Lam B. Use of AlloDerm in primary nipple reconstruction to improve long-term nipple projection. Plast Reconstr Surg 2007;119:1663-8. [Crossref] [PubMed]
- Nahabedian MY. Secondary nipple reconstruction using local flaps and AlloDerm. Plast Reconstr Surg 2005;115:2056-61. [Crossref] [PubMed]
- Evans KK, Rasko Y, Lenert J, et al. The Use of Calcium Hydroxylapatite for Nipple Projection After Failed Nipple-Areolar Reconstruction. Ann Plast Surg 2005;55:25-9. [Crossref] [PubMed]
- Yanaga H. Nipple-Areola Reconstruction with a Dermal-Fat Flap. Plast Reconstr Surg 2003;112:1863-9. [Crossref] [PubMed]
- Kim EK, Lee TJ. Use of Lyophilized Allogeneic Costal Cartilage. Ann Plas Surg 2011;66:128-30. [Crossref] [PubMed]
- Tierney BP, Hodde JP, Changkuon DI. Biologic collagen cylinder with skate flap technique for nipple reconstruction. Plast Surg Int 2014;2014:194087. [Crossref] [PubMed]
- Collins B, Williams JZ, Karu H, et al. Nipple Reconstruction with the Biodesign Nipple Reconstruction Cylinder: A Prospective Clinical Study. Plast Reconstr Surg Glob Open 2016;4:e832. [Crossref] [PubMed]
- Panettiere P, Marchetti L, Accorsi D. Filler injection enhances the projection of the reconstructed nipple: an original easy technique. Aesthetic Plast Surg 2005;29:287-94. [Crossref] [PubMed]
- Sue GR, Seither JG, Nguyen DH. Use of hyaluronic acid filler for enhancement of nipple projection following breast reconstruction: An easy and effective technique. JPRAS Open 2020;23:19-25. [Crossref] [PubMed]
- Serre A, Guillier D, Moris V, et al. Augmentation de la projection du mamelon dans les reconstructions mammaires par injection de derme artificiel. Ann Chir 2017;62:625-9. [Crossref] [PubMed]
- Wong RK, Wichterman L, Parson SD. Skin sparing nipple reconstruction with polytetrafluoroethylene implant. Ann Plast Surg 2008;61:256-8. [Crossref] [PubMed]
- Jankau J, Jaśkiewicz J, Ankiewicz A. A new method for using a silicone rod for permanent nipple projection after breast reconstruction procedures. Breast 2011;20:124-8. [Crossref] [PubMed]
- GC Aesthetics® Patient Video Testimonials. FixNip™ NRI is the final surgical step for Total Breast Reconstruction™ Nipple-Areola Complex Reconstruction Implant. April 2022. Internal Data.
- Vernice NA, Caughey S, Berri N, et al. Off-the-Shelf Nipple Engineering: Neonipple Formation via Implantation of Scaffolded Decellularized Ovine Xenograft. Ann Plast Surg 2022;88:S302-8. [Crossref] [PubMed]
- Samadi A, Premaratne ID, Wright MA, et al. Nipple Engineering: Maintaining Nipple Geometry with Externally Scaffolded Processed Autologous Costal Cartilage. J Plast Reconstr Aesthet Surg 2021;74:2596-603. [Crossref] [PubMed]
- Dong X, Premaratne ID, Sariibrahimoglu K, et al. 3D-printed poly-4-hydroxybutyrate bioabsorbable scaffolds for nipple reconstruction. Acta Biomater 2022;143:333-43. [Crossref] [PubMed]
- Thomas DJ. 3D bioprinting as a solution for engineering the nipple areola complex for breast cancer reconstruction. Int J Surg 2017;41:14-5. [Crossref] [PubMed]
- Oganesyan RV, Lellouch AG, Acun A, et al. Acellular Nipple Scaffold Development, Characterization, and Preliminary Biocompatibility Assessment in a Swine Model. Plast Reconstr Surg 2023;151:618e-29e. [Crossref] [PubMed]
- Pashos NC, Scarritt ME, Eagle ZR, et al. Characterization of an Acellular Scaffold for a Tissue Engineering Approach to the Nipple-Areolar Complex Reconstruction. Cells Tissues Organs 2017;203:183-93. [Crossref] [PubMed]
- Johnson PC, Klein GL. Orthotopic grafting of decellularized human nipple: Setting the stage and putative mechanism of healing. Breast 2020;54:96-8. [Crossref] [PubMed]
- Caronna VC, Rosenberg AF, Graham DM, et al. Viability of acellular biologic graft for nipple-areolar complex reconstruction in a non-human primate model. Sci Rep 2021;11:15085. [Crossref] [PubMed]
- Pashos NC, Graham DM, Burkett BJ, et al. Acellular Biologic Nipple-Areolar Complex Graft: In Vivo Murine and Nonhuman Primate Host Response Evaluation. Tissue Eng Part A 2020;26:872-85. [Crossref] [PubMed]